Lesson 3: Molecular Shape
Part d: Hybridized Orbital Theory
Part 3a:
Valence Shell Electron Pair Repulsion Theory (VSEPR)
Part 3b:
Advanced VSEPR
Part 3c:
Molecular Polarity
Part 3d: Hybridized Orbital Theory
A Geometry Problem
In our discussion of Valence Shell Electron Pair Repulsion (VSEPR) theory, we described how the valence shell electrons pairs on atoms spread out in three-dimensional space to establish well-defined geometries. These electron pairs are present as lone pairs and as shared pairs (bonds). The lone pairs reside in orbitals of a single atom. The shared pairs reside in orbitals of two atoms that overlap upon approach.
VSEPR predicts that the bonds resulting from the shared pairs make well-defined angles with one another. These angle measures are unique to the specific geometry. For example, a trigonal planar geometry has 120° bond angles and a tetrahedral geometry has 109.5° bond angles. And this is a problem. There are no orbitals in an atom that are spaced 109.5° apart or even 120° apart. The p orbitals of an atom are aligned along the axes at 90° angles to one another. If the central atom were to overlap its p orbitals with another atom and share electrons, then we would expect 90° bond angles. How can this geometry problem be solved?
Hybridization
In 1931, American chemist Linus Pauling proposed a concept known as orbital hybridization. Based on Schrodinger’s wave mechanical model, Pauling proposed that atoms hybridized their atomic orbitals upon close approach to another atom with which it would share electrons. Hybridization involves mixing valence shell s and p orbitals in varying ratios to produce same shape, equal energy, optimally spaced hybrid orbitals. Using Schrodinger’s wave equation, Pauling was able to show that such hybridizations could produce a set of hybrid orbitals with geometries consistent with the VSEPR predictions. We will describe his hybridization theory and then use it to explain the bonding in several example molecules.
Let’s consider the case of mixing one s orbital with all three p orbitals. Mixing four atomic orbitals results in four hybridized orbitals. Unlike the atomic orbitals from which they were formed, the hybridized orbitals all have the same shape and energy level. The diagram below is a graphical means of depicting the process. The resulting orbitals are referred to as sp3 hybrid orbitals. As we will see, this notation indicates that one s and three p atomic orbitals have been mixed to form four sp3 hybrid orbitals.
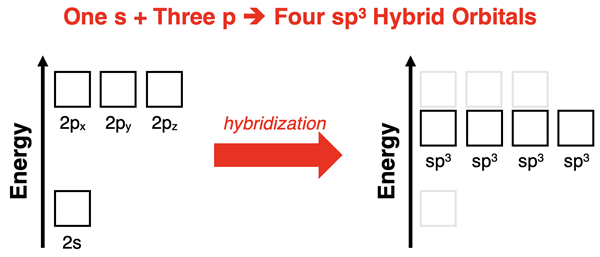
Assuming the mixing resulted in orbitals that had 25% s orbital character and 75% p orbital character, Pauling used the wave mechanical equation to compute the shapes and spatial orientation of these hybrid orbitals. Unlike the p orbitals that had two equal sized lobes and unlike the s orbitals that were spherical and symmetric about the origin, the sp3 hybrid orbitals had two lobes with one considerably larger than the other. The smaller lobe is typically ignored in most representations as it is assumed that the large lobe is the one that overlaps with the orbitals of terminal atoms.
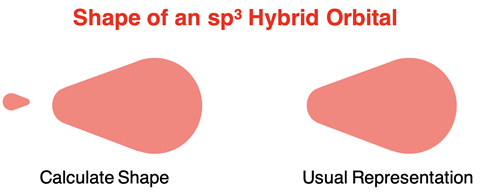
Pauling’s calculations showed that the sp3 orbitals formed a tetrahedral geometry with 109.5° angles between the lobes. These calculations satisfied the requirements of VSEPR theory and provided the first solution to the geometry problem. Any sp3 orbital holding two electrons would serve as the house for a lone pair. And any sp3 orbital holding a single electron would overlap with the orbital of a terminal atom to form a bond.
The sp3 hybridization addresses all situations in which the central atom of a molecule has four electron pairs, whether lone or shared pairs. But what is the solution for a molecule in which the central atom is surrounded by three electron pairs (or groups)? Pauling’s solution was to mix one s orbital with two p orbitals to form three sp2 hybrid orbitals. Pauling assumed the resulting hybrid would have 33% s character and 67% p character and relied on the Schrodinger wave equation to compute the shapes and spatial orientation of the sp2 hybrid orbitals. The resulting sp2 orbitals were positioned in a plane with 120° angles between their lobes. The resulting calculations were sufficient to explain the bonding any molecule with trigonal planar electron pair geometry.
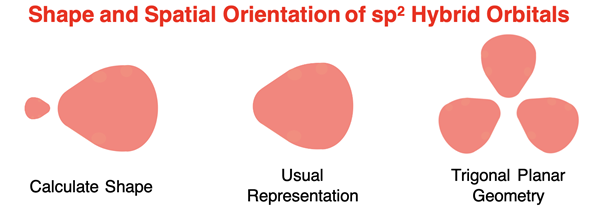
The mixing of one s orbital with two p orbitals to produce three sp2 hybrid orbitals leaves an unhybridized p orbital. This unhybridized p orbital is aligned perpendicular to the plane formed by the three sp2 orbitals and has an energy that is higher than the sp2 orbitals. Electron filling for hybridized orbitals follow the same rules as atomic orbitals – lower energy orbitals must completely fill before electrons enter higher energy orbitals. So, depending on the number of valence electrons, this unhybridized p orbital may or may not have electrons. It the p orbital has no electrons, then it simply does not involve itself in bonding. If it has electrons, it does involve itself in bonding. We discuss this topic below in the Double Bonds and Triple Bonds section.
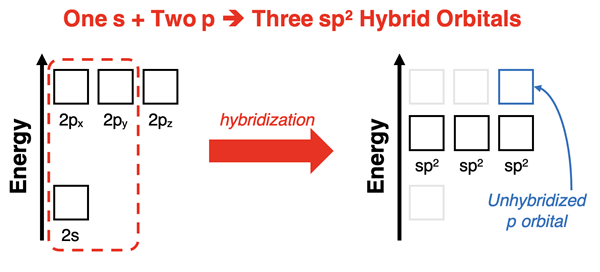
If there are only two electron pairs (or groups) about the central atom, then it will mix one s orbital with one p orbital to form two hybrid sp orbitals. These two hybrid orbitals are aligned along a line, making them perfect for accommodating linear electron pair geometries.
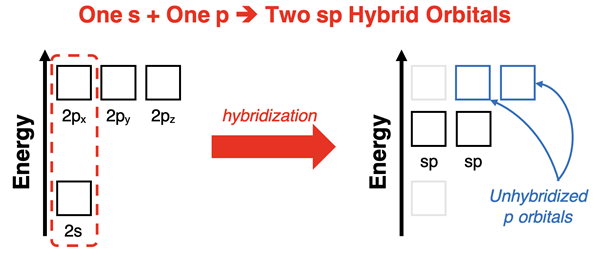
The hybridization of the s orbital with one p orbital leaves two unhybridized p orbitals. Let’s pretend for a moment that the central atom has x-y-z coordinate axes piercing its middle. In this case, we might think of the two sp hybrid orbitals being aligned along one of the axes – for example, along the x-axis. There will be two unhybridized p orbitals aligned along each of the other two axes. These orbitals may or may not be occupied by electrons. If they are, then they will participate in double or triple bonding.
Explaining CH4 Bonding
The central atom of a molecule will hybridize its atomic orbitals as it participates in bonding. The hybridization occurs so as to produce identically shaped orbitals with equal energy and the appropriate
orientation that minimizes valence shell electron pair repulsion while allowing for bonding to terminal atoms. For CH4, the Lewis electron dot diagram indicates four electron pairs around the central carbon. This requires sp3 hybrid orbitals aligned at 109.5° bond angles.
Carbon has four valence electrons. Each electron half-fills an sp3 hybrid orbital. Hydrogen has one valence electron and needs one more electron to satisfy the duet rule. Since there is one electron on hydrogen, it does not hybridize orbitals. Rather, hydrogen uses its 1s atomic orbital for bonding. Each of four hydrogen atoms overlaps its s orbital with one of the four sp3 hybrid orbitals of carbon. Carbon supplies one electron and hydrogen supplies one electron. Electron sharing allows carbon to satisfy the octet rule and hydrogen to satisfy the duet rule. The resulting molecule has tetrahedral electron pair geometry and tetrahedral molecular geometry.
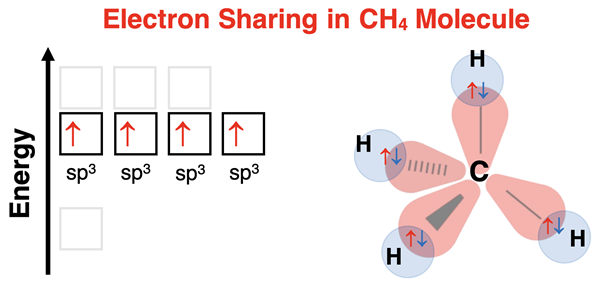
Explaining NH3 Bonding
Predicting the hybridization that occurs always begins with a Lewis electron dot diagram. The dot diagram shows the number of bonds and lone pairs on the central atom in the molecule. Hybridization occurs to accommodate the needs of the atom to have equivalent orbitals with equal energy and an orientation that minimizes valence shell electron pair repulsion while allowing for bonding to terminal atoms. For NH3, the Lewis electron dot diagram indicates four electron pairs around the central carbon. This requires sp3 hybrid orbitals. The Lewis diagram shows three electron pairs being shared with hydrogen and one lone pair.
Nitrogen has five valence electrons. With five electrons, three of the sp3 hybrid orbitals will be half-filled with electrons and one sp3 hybrid orbital will be filled with two electrons. Hydrogen has one valence electron and needs one more electron to satisfy the duet rule. Each of three hydrogen atoms overlaps its s orbital with one of the three sp3 hybrid orbitals of nitrogen. Nitrogen supplies one electron from its half-filled sp3 orbitals and hydrogen supplies one electron. Electron sharing allows nitrogen to satisfy the octet rule and hydrogen to satisfy the duet rule. The one remaining sp3 hybrid orbital of nitrogen houses the lone pair. The resulting molecule has tetrahedral electron pair geometry. Because of the lone pair on nitrogen, the molecular geometry will be trigonal pyramidal.
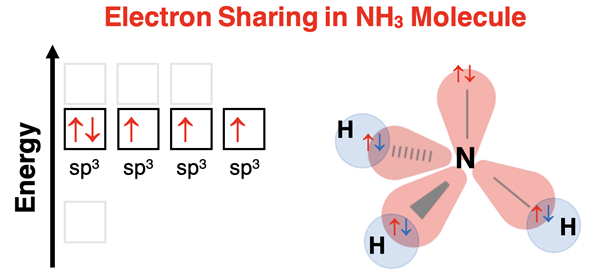
Explaining BH3 Bonding
The electron dot diagram for boron trihydride (BH3) is shown at the right. Boron is a rare case of an element that seldom acquires an octet of valence shell electrons. There are three electron pairs surrounding the central boron. Boron will undergo sp2 hybridization to accommodate its bonding requirements of having three same-shaped orbitals with equal energy and proper orientation. Boron leaves one of its p orbitals unhybridized. This orbital does not participate in bonding.
Boron has three valence electrons. With three electrons, all three of its sp2 hybrid orbitals are half-filled with electrons. Hydrogen has one valence electron and needs one more electron to satisfy the duet rule. Each of three hydrogen atoms overlaps its s orbital with one of the three sp2 hybrid orbitals of boron. Boron supplies one electron from its half-filled sp2 orbitals and hydrogen supplies one electron. Electron sharing occurs and three single B-H bonds are formed. The resulting molecule has trigonal planar electron pair geometry and trigonal planar molecular geometry.
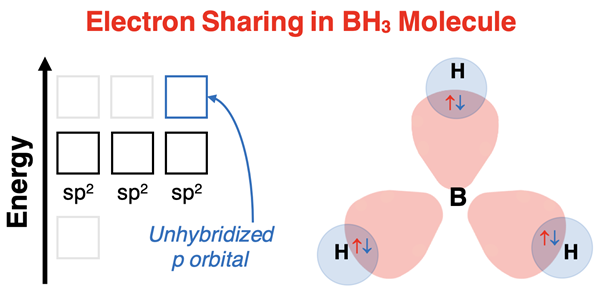
Expanding the Octet
In Lesson 2, situations in which atoms expand their octet were discussed. The PCl5 molecule was an example of a central atom with five shared electron pairs for a total of 10 valence electrons. The SF6 molecule was another example of a situation involving a central atom exceeding an octet of electrons. For this to occur, the element of the central atom must be in period 3 or lower of the periodic table. The n=3 energy shell is the lowest energy electron shell that has d orbitals. The d orbitals are needed to house the additional electrons beyond the eight that the s and p orbitals can house.
The use of d orbitals to house electrons on the central atom means that d orbitals need to be involved in hybridization. Mixing one d orbital with an s orbital and three p orbitals from the same energy level will result in the formation of five sp3d hybrid orbitals. Calculations show that the resulting orbitals assume a trigonal bipyramidal geometry. Three of the hybrid orbitals are arranged in a triangular plane with 120° angles between them and two of the hybrid orbitals are oriented perpendicular to the plane (above and below it).
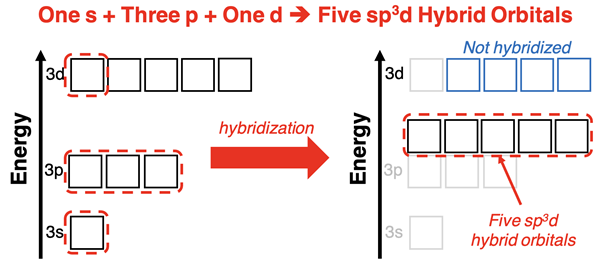
Mixing two d orbitals with an s orbital and three p orbitals from the same energy level will result in the formation of five sp3d2 hybrid orbitals. Calculations show that the resulting orbitals assume an octahedral geometry.
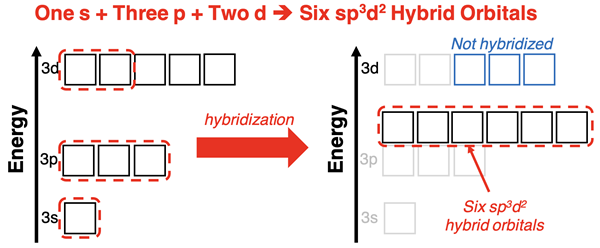
Explaining PCl5 Bonding
The electron dot diagram for phosphorus pentachloride (PCl5) is shown at the right. Phosphorus is exceeding 8 electrons and thus must hybridize d orbitals with s and p orbitals to accommodate five electron pairs. The result is a collection of five sp3d hybrid orbitals having the same energy and assume a trigonal bipyramidal geometry. Phosphorus has five valence electrons which half-fill each of the hybrid orbitals. Chlorine also hybridizes its s and p orbitals to form sp3 hybrid orbitals. Three of chlorine’s hybrid orbitals contain lone pairs and the fourth contains a single electron. It is this fourth sp3 orbital on chlorine that overlaps with the half-filled sp3d hybrid orbitals of phosphorus. Five P-Cl bonds form. The resulting molecule has trigonal bipyramidal electron pair geometry and trigonal bipyramidal molecular geometry.

Explaining SF6 Bonding
The electron dot diagram for sulfur hexafluoride (SF6) is shown at the right. The manner in which sulfur hybridizes is determined by its electron pair requirements. Sulfur needs sufficient orbitals to house six electron pairs, all involved in bonding with a fluorine atom. Sulfur hybridizes two d orbitals with one s and three p orbitals to accommodate these six electron pairs. The result is a collection of six sp3d2 hybrid orbitals having the same energy and assuming an octahedral geometry. Sulfur has six valence electrons which half-fill each of the six hybrid orbitals. Fluorine hybridizes its s and p atomic orbitals to form sp3 hybrid orbitals. Three of its hybrid orbitals contain lone pairs and the fourth contains a single electron. It is this fourth sp3 orbital on fluorine that overlaps with the half-filled sp3d2 hybrid orbitals of sulfur. Six S-F bonds are formed. The resulting molecule has octahedral electron pair geometry and octahedral molecular geometry.
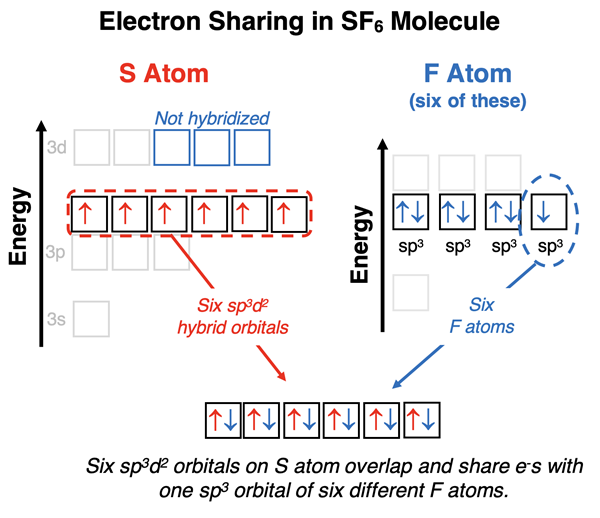
Explaining SF4 Bonding
The electron dot diagram for sulfur tetrafluoride (SF4) is shown at the right. Observe the five electron pairs on sulfur; one is a lone pair and the other four are shared pairs. Because sulfur needs five hybrid orbitals, it hybridizes one d orbital with one s and three p orbitals to accommodate these five electron pairs. The result is a collection of five sp3d hybrid orbitals having the same energy and assuming a trigonal bipyramidal geometry. Sulfur has six valence electrons which half-fill four of the sp3d orbitals and fully fill one of the sp3d orbital. The half-filled sp3d hybrid orbitals will participate in bonding with the four fluorine atoms and the one fully filled sp3d orbital will house the lone pair. Fluorine hybridizes its s and p atomic orbitals to form sp3 hybrid orbitals. Three of its hybrid orbitals contain lone pairs and the fourth contains a single electron. It is this fourth sp3 orbital on fluorine that overlaps with the half-filled sp3d hybrid orbitals of sulfur. Four S-F bonds are formed and one lone pair resides on the sulfur. The lone pair on sulfur occupies one of the sp3d hybrid orbitals in the triangular plane. The resulting molecule has trigonal bipyramidal electron pair geometry and seesaw molecular geometry.
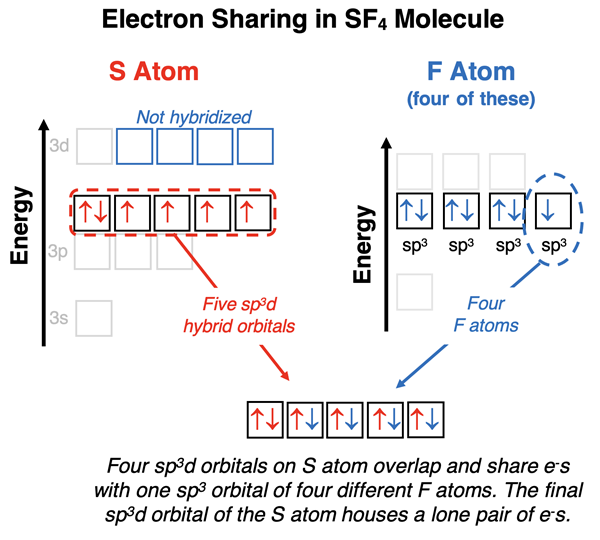
Double Bonds and Triple Bonds
Each molecule so far has included single-bond atoms. We have not yet seen how multiple (double and triple) bonds form. Single bonds result from the head-on orbital overlap of approaching atoms. Such overlaps are referred to as sigma overlaps or sigma bonds (abbreviated in Greek as σ). The overlap of orbitals occurs along the bond axis (imaginary line that connects the nuclei of the two bonded atoms). These sigma overlaps occur between hybrid orbitals on the central atom (A) and hybrid orbitals on the terminal atom (X). (The H atom uses its s orbital for these overlaps.)
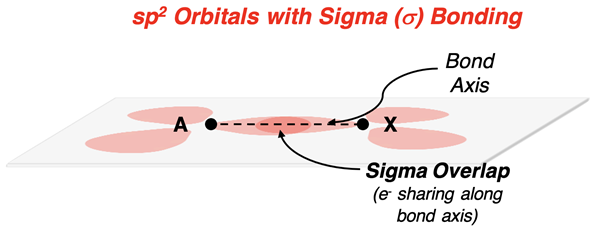
Double and triple bonds are formed by side-to-side overlaps of p orbitals. Atoms reserve a p orbital for double bonds and hybridize the s orbital with the remaining p orbitals (if needed). The p orbitals are oriented perpendicular to the bond axis connecting the nuclei of the bonded atoms. As the atoms approach, the p orbitals also approach. When close enough, there is significant enough interaction between atoms that electrons are shared by the two p-orbital lobes of the two atoms. The electron density above and below the bond axis increases. This is referred to as a pi overlap or pi bond (abbreviated in Greek as π). Every double bond consists of a sigma overlap of hybrid orbitals and a pi overlap of unhybridized p orbitals.
Atoms reserve two p orbitals for triple bonds and hybridize the s and remaining p orbital for the sigma overlap. The unhybridized p orbitals are perpendicular to the bond axis but in different planes to one another. They each undergo pi orbital overlap. Every triple bond consists of one sigma overlap and two pi overlaps of unhybridized p orbitals.
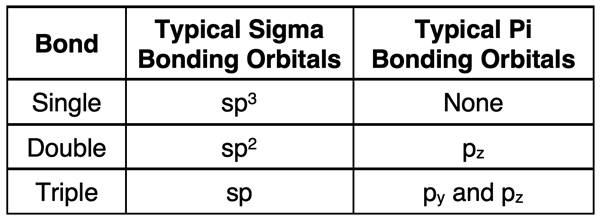
Explaining O2 Bonding
The O2 molecule provides a simple example of the involvement of orbitals in double bonding. The electron dot diagram is shown. There are two lone pairs on each oxygen atom and a double bond between them. Each oxygen atom will need to reserve a p orbital for the pi overlap above and below the bond axis. The s orbital and remaining two p orbitals hybridize to form three sp2 orbitals that have a trigonal planar arrangement. The lobes of the unhybridized p orbitals extend above and below the plane. For each oxygen atom, two of the sp2 hybrid orbitals are used to house the lone pairs. The remaining sp2 orbital stretches along the bond axis and undergoes a sigma overlap. The unhybridized p orbitals undergo a pi overlap above and below the plane. The combination of sigma overlap and pi overlap is the double bond.
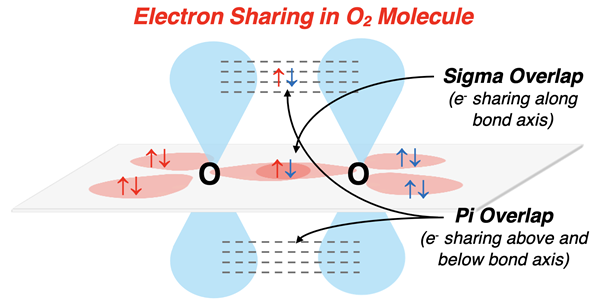
Explaining N2 Bonding
The N2 molecule provides a good example of the involvement of orbitals in triple bonding. The electron dot diagram is shown. Each nitrogen has one lone pair and three shared pairs to form a triple bond. A triple bond requires two unhybridized p orbitals for each nitrogen atom. The remaining p orbital hybridizes with the s orbital to form two sp hybrid orbitals with a linear arrangement. The p orbitals are perpendicular to the linearly aligned sp orbitals. For each nitrogen atom, one of the sp hybrid orbitals is used to house the lone pair. The other sp orbital stretches along the bond axis and undergoes a sigma overlap. The two sets of unhybridized p orbitals undergo pi overlaps above and below the bond axis and in front and behind the bond axis. The combination of the sigma overlap and the two pi overlaps is the triple bond.
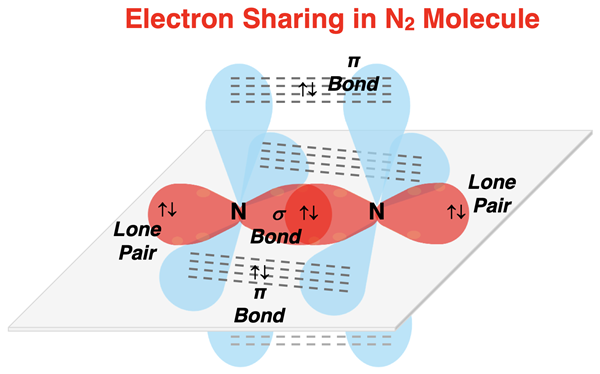
Explaining C2H4 Bonding
Let’s consider the C2H4 molecule for a more complicated instance of multiple bonds. The electron dot diagram is shown at the right. Each carbon atom forms two single bonds with two hydrogen atoms and one double bond with the other carbon atom. There are no lone pairs. The double bond requires that the carbon reserve a p orbital for a pi overlap and then hybridize two p orbitals with an s orbital to form three sp2 hybrid orbitals. The sp2 hybrid orbitals are aligned in a plane and the p orbitals are perpendicular to the plane. Each hydrogen atom supplies one electron in a spherical s orbital; it only needs an additional electron to satisfy its duet rule. Each hydrogen atom will share an electron pair with two of carbon’s sp2 hybrid orbital. The third sp2 orbital on carbon that stretches along the bond axis will undergo a sigma overlap with the carbon orbital on the other carbon. The p orbitals that are perpendicular to the plane of the sp2 orbitals will undergo a pi overlap.
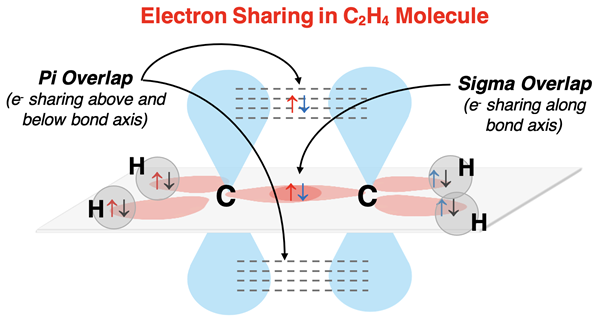
Beyond Localization of Electrons
Lesson 2 and Lesson 3 of Chapter 6 have focused on covalent bonding in molecules. There have been three major models presented that work together to explain the intricate nature of the bonds and lone pair electrons. The models are 1) Lewis electron dot diagram, 2) Valence Shell Electron Pair Repulsion theory, and 3) Hybridized Orbital theory. All three models are localized electron models. They presume that electrons are occupied on atoms or between atoms. The models do not entertain the idea that electrons can be delocalized across several atoms. This limitation becomes particularly problematic as we attempt to describe the electron sharing in resonance structures.
Resonance structures were introduced in Lesson 2c. Sulfur dioxide, SO2, is an example of a molecule that exhibits resonance. We can imagine it existing as one of two equivalent electron dot diagrams.

Both diagrams infer that there are two types of S-O bonds in the molecule – an S-O single bond and an S-O double bond. Laboratory analysis of the molecule indicates that there is only one type of bond and that it has properties (bond length and bond energy) that are intermediary between an S-O single bond and an S-O double bond. The localized electron models do not have a good explanation for this.
Thinking in terms of delocalized electrons allows one to provide an explanation for the bonding in SO2. Our localized electron model would analyze the dot diagram at the right and suggest the following conventional thought:
- Central S atom: three sp2 hybrid orbitals (one lone pair, two sigma overlaps) and one p orbital perpendicular to the plane for pi overlap.
- Left O atom: four sp3 hybrid orbitals (three lone pairs and one sigma overlap with central S atom)
- Right O atom: three sp2 hybrid orbitals (two lone pairs, one sigma overlap) and one p orbital perpendicular to the plane for pi overlap.
Since both S-O bonds are observed to be identical, let’s depart from the conventional (above) and suggest that both O atoms use the same type of hybridization – sp
2 hybrid orbitals with a p orbital reserved for pi overlap. The p orbitals of both O atoms and the S atom will sit above the plane of the set of sp
2 hybrid orbitals. Two electrons will be delocalized across all three atoms instead of being localized between two atoms. The delocalized electron adjustment to the usual model simply pictures these electrons as being involved in
pi bonding as opposed to being a double bond. The adjustment provides a great explanation of resonance structures and is consistent with empirical observations of their being only one type of bond between the S and the O atoms.
A Summary of Hybrid Atomic Orbitals
The hybrid orbital theory represents the chemist’s effort to provide orbitals with the proper geometry to accommodate the pairs of electrons on the central atom (and terminal atoms). Determining exactly how an atom will hybridize requires that an electron dot diagram be constructed. Once constructed, the number of electron groups surrounding the central atom can be determined. Atoms will hybridize in order to generate orbitals with the proper geometry to accommodate the lone pairs and participate in bonding. The number of hybrid orbitals that are formed is equal to the number of atomic orbitals that are mixed. The table below summarizes the relationships discussed on this page.
Before You Leave
- Download our Study Card on Hybrid Orbitals. Save it to a safe location and use it as a review tool.
- The Check Your Understanding section below include questions with answers and explanations. It provides a great chance to self-assess your understanding.
Check Your Understanding
Use the following questions to assess your understanding. Tap the Check Answer buttons when ready.
1. How does the number of atomic orbitals that are hybridized compare to the number of hybrid orbitals that are produced?
a. They are always the same. Guaranteed!
b. There are less hybrid orbitals formed than the number of atomic orbitals that are hybridized.
c. There are more hybrid orbitals formed than the number of atomic orbitals that are hybridized.
d. It’s just not that straight forward of a comparison. The answer depends on a number of variables – like the actual atom, whether there are double or triple bonds, whether the d orbitals are involved or not, which row of the periodic table the element is in, and even whether the molecule is diatomic, triatomic, or other-atomic.
2. What atomic orbitals and how many are hybridized to form …
- sp hybrid orbitals.
- sp2 hybrid orbitals.
- sp3 hybrid orbitals.
- sp3d hybrid orbitals.
- sp3d2 hybrid orbitals.
3. The diagrams show the electron pairs around the central atom (
A).
For each, identify the electron pair geometry and the hybridization of the central atom.
4. Electron dot diagrams are shown below. For each diagram, describe the bonding that occurs between the central atom and the terminal atoms.
5. For the following molecules:
- Draw the electron dot diagram.
- Identify the electron pair geometry.
- Identify the hybridization of the central atom and the terminal atoms.
- Describe the bonding that occurs.
- CCl4
- BCl3
- OCl2
- CO2
6. The carbonate ion, CO32-, demonstrates resonance. Three resonance structures are shown below. Use the concept of delocalization to describe the hybridization of each atom and the bonding occurring among atoms.
